Search


Our new publication in Molecules "Recent Configurational Advances for Solid-State Lithium Batteries"
Solid-state lithium metal batteries offer superior energy density, longer lifespan, and enhanced safety compared to traditional...
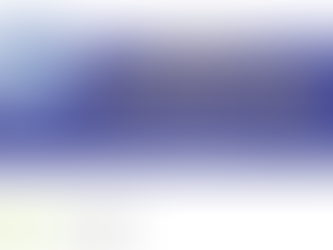
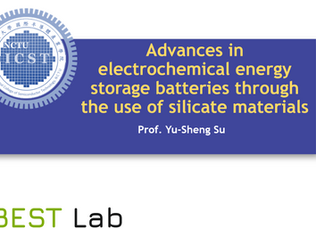
Prof. Su gave a talk at Purdue-NYCU Joint Workshop
Purdue-NYCU Joint Workshop on April 27, 2023 Location: Birck Nanotechnology Center 1001 Title of the workshop “Advanced Nanoelectronics...
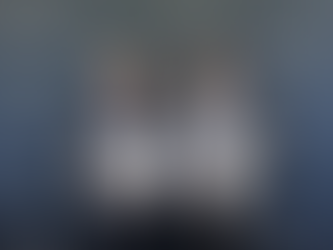
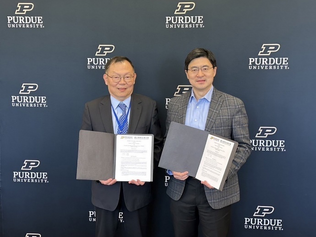
NYCU/Purdue: Signing Student Exchange Agreement and MoU for Online Course Program
A new chapter was opened when President Mung Chiang of Purdue University and President Chi-Hung Lin of National Yang Ming Chiao Tung...
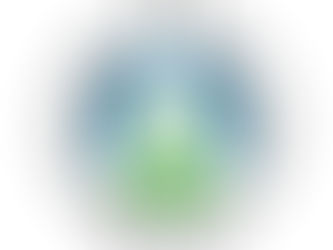
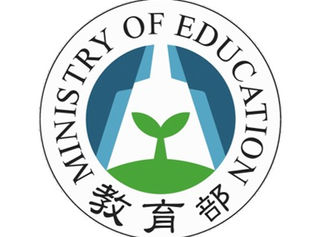
Congratulations to Yue-Sheng Chen for his MOE Doctoral Study Abroad Scholarship
Congratulations to Yue-Sheng Chen for his Doctoral Study Abroad Scholarship (The University of Sydney)! https://depart.moe.edu.tw/ED2500/...