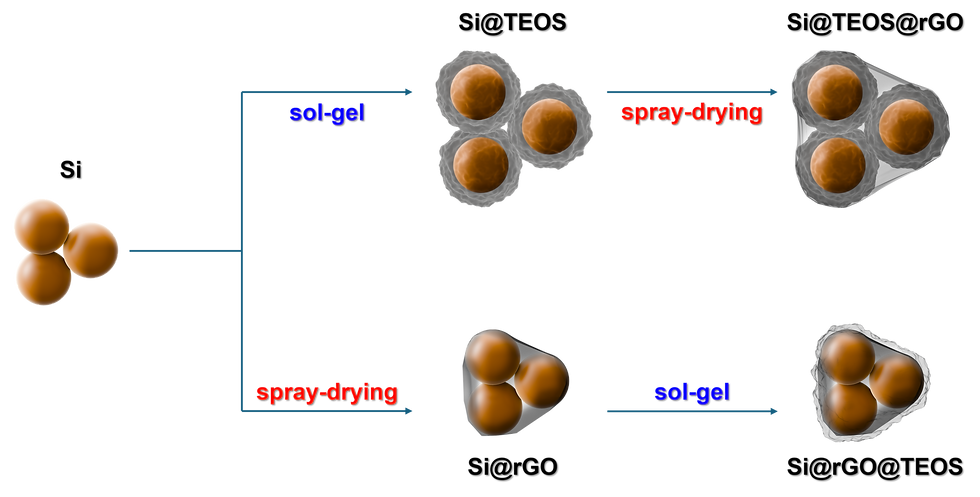
This study explores the optimization of double-shelled silicon (Si) composite anodes for lithium-ion batteries (LIBs) by examining the coating sequence of tetraethyl orthosilicate (TEOS) and reduced graphene oxide (rGO). The Si55@TEOS29@rGO16 anode, which features an inner TEOS-derived layer and outer rGO shell, exhibits an initial capacity of 1763 mA h g−1 at 0.1C and maintains 1153 mA h g⁻¹ after 300 cycles at 0.5C, achieving a capacity retention of 85.5% when incorporating the trimethylsilyl phosphate (TMSPi) electrolyte additive. The performance improvement is attributed to the hierarchical structure, where the porous SiO2 layer effectively passivates the Si core, while the rGO shell enhances conductivity and structural stability. Comparative analysis reveals that the optimized coating sequence and TEOS content significantly enhance cycling stability, with the Si55@TEOS29@rGO16 anode surpassing other configurations in specific capacity after 129 cycles. Scanning electron microscopy (SEM) and transmission electron microscopy (TEM) analyses confirm the uniform coating and structure stability after cycling. Electrochemical impedance spectroscopy (EIS) shows reduced impedance, and cyclic voltammetry (CV) indicates stable lithiation/delithiation processes. The inclusion of the TMSPi additive further stabilizes the solid electrolyte interphase (SEI) and inhibits capacity fading during initial cycles. This study demonstrates that optimizing the coating sequence, SiO2 content, and electrolyte additives can significantly improve the electrochemical performance and durability of Si-based anodes, positioning them as strong candidates for next-generation LIBs.
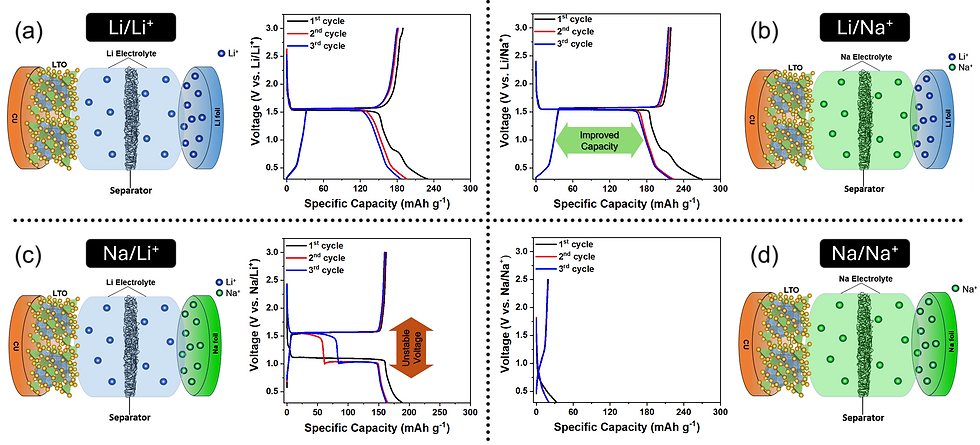
Li4Ti5O12 (LTO) is a highly promising anode material for both Li-ion and Na-ion batteries due to its remarkable structural stability, minimal volumetric expansion, and high safety. Despite extensive research on LTO performance in conventional systems, little attention has been given to its behavior in mismatched electrolyte environments. This study explores the electrochemical behavior of LTO anodes in Na⁺-containing electrolytes for Li⁺/Na+ intercalation, providing new insights into ion intercalation mechanisms and enhancing battery performance. Using different counter electrode and electrolyte configurations, we reveal that LTO exhibits superior capacity and cycle stability in a mismatched Li/Na⁺ environment compared to the standard Li/Li⁺ system, delivering capacities of up to 219 mAh g−1. Electrochemical and structural analyses indicate that enhanced performance stems from the unique interaction between Li⁺/Na⁺ and LTO structure, leading to improved charge-discharge performance. Additionally, the Na/Li⁺ configuration displayed unique behavior, where a distinct plateau splitting emerged after the first cycle, indicating complex ion interactions that ultimately affect cycling stability. These findings not only highlight the potential of LTO anodes in mismatched electrolyte systems but also offer a pathway toward more cost-effective and sustainable energy storage solutions by leveraging sodium-ion electrolytes in place of traditional lithium-based systems.
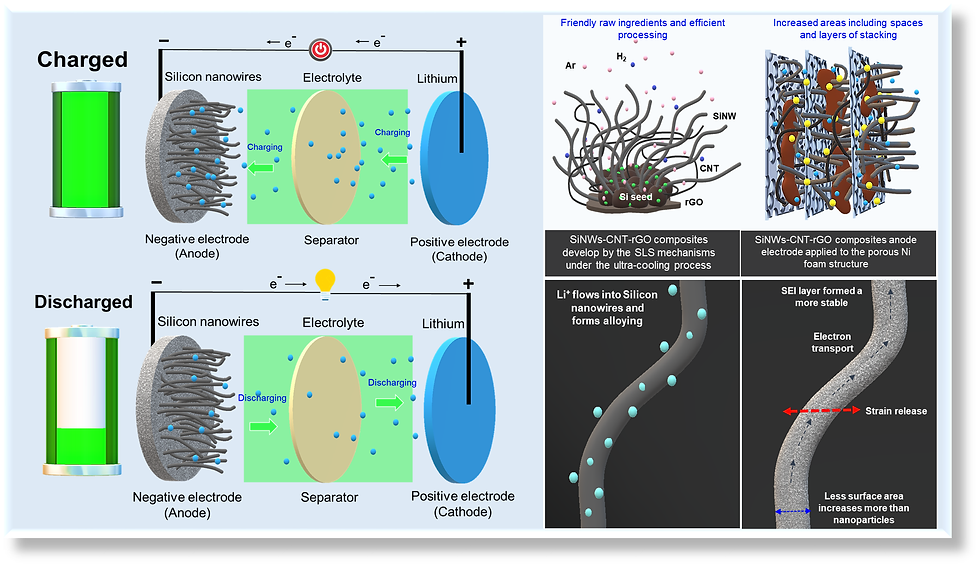
Silicon nanowires (SiNWs) address key challenges associated with traditional silicon anodes in lithium-ion batteries (LIBs) by mitigating pulverization and providing efficient ion transport pathways. However, their high fabrication cost and poor structural stability remain significant obstacles. This study presents a novel SiNW-CNT-rGO composite fabricated via a solid-liquid-solid (SLS) growth mechanism using NiO/Ni catalysts to establish a 3D conductive network. The composite integrates carbon nanotubes (CNTs) and reduced graphene oxide (rGO) to enhance electrical conductivity and stabilize the solid electrolyte interphase (SEI). Embedding the composite in 3D nickel foam (NF) further improves structural integrity and enhances Li+ ion diffusion, outperforming conventional copper foil (CF) current collectors in capacity across various rates. The integrated design provides efficient electron and ion transport pathways while mitigating mechanical stresses. Electrochemical evaluations reveal that the SiNW-CNT-rGO@NF anode significantly improves specific capacity, cycling stability, and rate capability compared to SiNW-based anodes with CF. These findings reveal the potential of the 3D SiNW-CNT-rGO@NF architecture as a scalable and cost-effective solution for next-generation high-performance LIBs.