The development of lithium-sulfur batteries (LSBs) marks a crucial milestone in advancing energy storage solutions essential for sustainable energy transitions. With high theoretical specific capacity, cost-effectiveness, and reduced ecological footprint, LSBs promise to enhance electric vehicle ranges, extend portable electronics' operational times, and stabilize grids integrated with renewable energy. However, challenges like complex processing, electrode instability, and poor cycling stability hinder their commercialization. This study introduces a novel battery design that addresses these issues by coating sulfur directly onto the separator instead of the current collector, demonstrating that active sulfur can be effectively utilized without being incorporated into the electrode structure. Using an interwoven substrate made from carbon nanotube (CNT) fabric adorned with reduced graphene oxide (rGO), this setup enhances manufacturing scalability, supports optimal sulfur utilization, and improves battery performance. The rGO decoration provides multiple highly conductive polysulfide trapping sites, enhancing active material reutilization, while the flexibility and mechanical strength of CNT fabric contribute to electrode integrity. This combination boosts electrical conductivity and polysulfide-capturing capability, effectively managing migrating sulfur species during charge-discharge cycles and mitigating sulfur loss and polysulfide shuttling. The results demonstrate superior cycling stability and efficiency, highlighting the potential of this approach in advancing LSB technology.
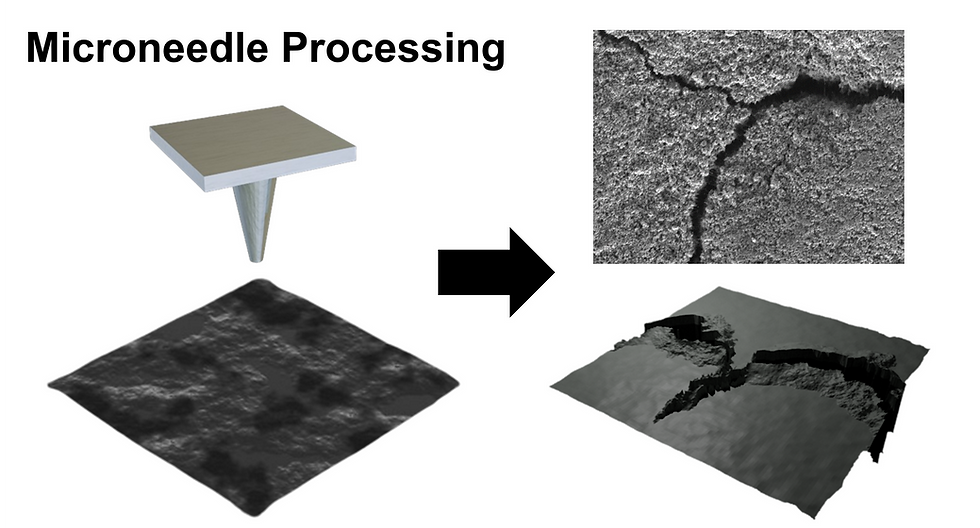
Advancing battery electrode performance is essential for high-power applications. Traditional fabrication methods for porous electrodes, while effective, often face challenges of complexity, cost, and environmental impact. Inspired by acupuncture, here we introduce aneco-friendly and cost-effective microneedle process for fabricating lithium iron phosphate electrodes. This technique employs commercial cosmetic microneedle molds to create low-curvature holes on electrode surfaces, significantly enhancing electrolyte infiltration and ion transport kinetics. The punctured electrodes were prepared and characterized, with comparisons to pristine electrodes conducted using scanning electron microscopy, 3D metallurgical microscopy, and detailed electrochemical evaluations. Our results show that the microneedle-processed electrodes exhibit superior rate performance and diffusion properties. Simulations and experimental data reveal that the low-curvature holes reduce Li-ion concentration polarization and improve Li-ion transport within the electrode. This enhancement leads to higher specific capacities and better rate capabilities in the punctured electrodes. The findings highlight the potential of this innovative microneedle technique for large-scale production of high-performance electrodes, offering a promising avenue for the development of high-power-density batteries.
The growing demand for cost-effective and sustainable energy storage solutions has spurred interest in novel anode materials for lithium-ion batteries (LIBs). This study explores the potential of small molecule polycyclic aromatic hydrocarbons (SMPAHs) as promising candidates for LIB anodes. Through a comprehensive experimental approach involving electrode fabrication, material characterization, and electrochemical testing, the electrochemical performance of SMPAHs, including naphthalene, biphenyl, 9,9-dimethylfluorene, phenanthrene, p-terphenyl, and pyrene, is thoroughly investigated. The results reveal the impressive cycle stability, high specific capacity, and excellent rate capability of the SMPAH electrode. Additionally, a direct contact prelithiation strategy is implemented to enhance the initial Coulombic efficiency (ICE) of SMPAH anodes, yielding significant improvements in the ICE and cycle stability. Computational simulations provide valuable insights into the electrochemical behavior and lithium storage mechanisms of SMPAHs, confirming their potential as effective anode materials. The simulations reveal favorable lithium adsorption sites, the predominant storage mechanisms, and the dissolution mechanism of pyrene through computational calculations. Overall, this study highlights the promise of SMPAHs as sustainable anode materials for LIBs, advancing energy storage technologies toward a greener future.